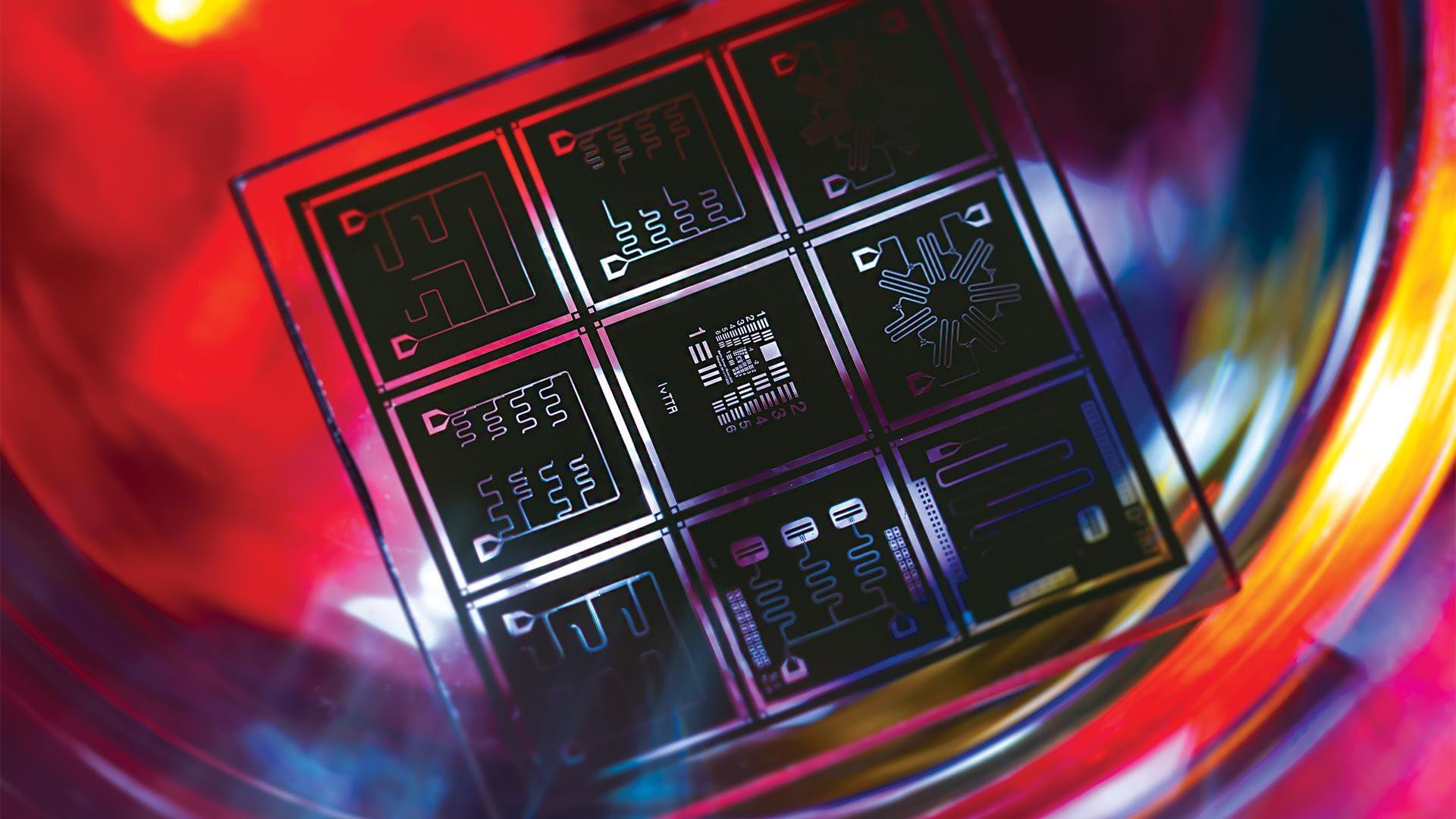
It’s hard to envision a time when computers didn’t more or less disappear into beige office landscapes or pile up, obsolete in closets like increasingly ancient geological strata. The technological behemoths that Franz Klein works with, however, still evoke a twinge of dawn-of-the-space-age wonder.
As a high-performance computing engineer in the University of Maryland’s Division of Information Technology, he helps run massive computing clusters linked by kilometers of cable that gobble enough electricity to run hundreds of average houses while crunching exponentially more data than your laptop. Astronomers, geneticists, climatologists and others queue up for solutions only supercomputers like UMD's soon-to-debut Zaratan, not to mention larger clusters at government laboratories and high-tech firms, can spit out.
Yet with all this digital horsepower at his fingertips, Klein now is venturing in a new direction with his recent concurrent appointment directing the National Quantum Lab at Maryland, aka the Q-Lab. Along with the National Science Foundation (NSF)-funded Quantum Leap Challenge Institute for Robust Quantum Simulation (RQS), it's one of two recent initiatives centered at UMD to accelerate the nascent field of quantum computing and help map out an unfamiliar new world. As various quantum technologies take off, a new class of computers enabled by the latest advances in physics is expected to revolutionize every aspect of life, just as today’s devices led to the internet, mobile technology and advanced manufacturing.
[Q-Lab Seed Grant Program to Support New Quantum Connections]
But this world of possibility has plenty of technological terra incognita, including limitations that echo the 1940s, when the first computers sparked to life, Klein saId.
“There’s a mismatch between the reality of the technology and what quantum computer science and information science want to do with large, ideal systems that don't exist yet,” he said.
UMD's aggressive new push, he adds, could help reality catch up.
Hardcore Engineering, ‘Wacky’ Ideas
Klein isn’t the first to note this mismatch, and the initiatives approach it from very different angles: The RQS, a multi-institutional project led by UMD and supported by $25 million from the NSF, takes a basic science tack with computer scientists, physicists, engineers and chemists exploring the universe at the scale of atoms while experimenting with different technologies and systems. It’s all connected by the interim step toward powerful quantum computers known as quantum simulation—using the current hardware to "simulate" and study quantum systems in nature, from the workings of molecules to how light behaves.
The Q-Lab, which is expected to open its doors this fall in the university’s Discovery District with a $20 million investment from UMD, is a partnership with university spinoff IonQ. The lab centers on the company’s campaign to translate discoveries that emerged from the Department of Physics into the first breakthrough quantum computer, establishing real-world viability for the technology. UMD researchers, partners and students, meanwhile, have access to the hardware and research opportunities that result from IonQ’s race with tech giants like IBM and Google.
“No other university in the United States is able to provide students and researchers this level of hands-on contact with commercial-grade quantum computing technology and insights from experts working in this emerging field,” University of Maryland President Darryll J. Pines said last fall in announcing the lab.
Both efforts draw on one of the largest quantum research efforts in the world at UMD. The university boasts eight related centers and institutes, a long-running partnership with the National Institute of Standards and Technology, and hundreds of researchers who delve into all aspects of quantum science and technology, from discovering new superconducting materials to exploring the mathematical and conceptual underpinnings of matter.
The foundations of the RQS and Q-Lab appear very different—“let’s see what we can find” science vs. “let’s reach the goal” engineering—because it’s impossible to predict how technological revolutions are born, said Alicia J. Kollár, a RQS co-investigator and Chesapeake Assistant Professor of Physics. For example, a 1950s experiment at Bell Labs resulted in the discovery of a transistor-manufacturing process that helped make modern computers possible, only after an apparent disaster. “They couldn’t figure it out, and then one day, their apparatus exploded—and then it worked,” she said.
“If you’re truly going to build a digital quantum computer, you can’t do it without hardcore engineering; you also need the wacky ideas and discoveries from doing science,” Kollár said.
Qubit Connection
A wide developmental gulf divides quantum computing from the “classical” computing that runs phones, smart fridges and supercomputers. With tech roots stretching back 80 years to the dawn of transistors, even a standard processor on sale at Best Buy can handle billions of “bits”-zeros and ones that are the basic units of information in standard computing. But quantum computers? You and some friends could count on your fingers how many “qubits,” or quantum bits, they have.
But thanks to non-intuitive quantum effects like “superposition,” which essentially allows a qubit to be both a zero and a one simultaneously, and “entanglement,” which means qubits can be correlated in classically impossible ways, each qubit far surpasses a bit in terms of information-processing potential.
A machine of just a few thousand qubits should outperform the biggest classical supercomputer: cracking modern cryptography, perhaps supercharging artificial intelligence and machine learning, or mastering chemistry at an unprecedented level.
But getting to 1,000 reliable qubits, for now, is as daunting as Everest before Hillary and Tenzing.
Qubits must maintain delicate quantum states for the computer to operate; errors result from vibrations, temperature fluctuations or other environmental variables. The upshot, many believe, is that each functional qubit will need many more backup qubits to correct errors, unless a more reliable qubit technology comes to dominate. So 1,000 “logical” qubits might translate into 10,000, or even 100,000 individual qubits.
Accessible Goals
But what if we dropped the requirement that a quantum computer be as reliable as a regular computer? What if we worked up to Everest by first climbing hills? Broadly, that describes the RQS’s approach, said its director and principal investigator, Andrew Childs, a professor of computer science who also co-leads the Joint Center for Quantum Information and Computer Science, a UMD-NIST partnership.
When quantum computers were first proposed in the 1980s, the idea was to use them to understand quantum systems too complex for classical computers, Childs said.
“One approach to that is you build a large-scale, fault-tolerant quantum computer that you can program any way you want to simulate quantum mechanics,” he said.
Since such computers don’t yet exist, scientists at Maryland have led the way with an alternative approach that is already feasible: analog quantum simulation. “In this case, instead of a digital computer you can use for anything, you build a system that will reproduce the features of the quantum system you want to study,” he said. “Maybe it’s somewhat programmable in that you have some knobs you twist to adjust the parameters, but it’s still mocking up a system rather than providing the complete flexibility of a full digital computer.”
Kollár, who is focusing on developing a new kind of superconducting qubit, calls herself “an analog hardware person at heart.”
“With a digital quantum computer, the goal is complete control, and the qubit does exactly what you want,” she said. “A quantum simulator is much more about letting nature run its course and figuring out what it’s doing—but trying to find an interesting course.”
Even a modest knob-twisting simulator, Childs points out, is still a quantum processor, even if it’s not what he envisions in his theoretical work on algorithms for ideal systems. “For now, it’s an accessible way to move forward … and at the same time, understand some of the big-picture questions.”
Expanding the Network
For applications beyond research, reliability is king. That’s an area in which IonQ has been generated big waves from its headquarters off Campus Drive. The company reports that its “trapped ion” technology—ytterbium and barium atoms suspended in a vacuum and controlled by lasers—creates a qubit that can remain stable for days, far longer than competitors’ superconductor-based qubits manage. As reported in a recent publication about creating fault tolerance in Nature, the technology is raising hopes that IonQ might lead the field past the crippling error problem.
So it’s remarkable that even UMD undergrads at the Q-Lab have been handed the keys to a system that can make a claim to being the world’s best—a level of institution-wide access that will continue to expand.
“It's going to push the state of the art,” said Ron Walsworth, director of UMD’s Quantum Technology Center (QTC) and a professor in engineering and physics who serves on the lab’s leadership committee. “Having a company develop technology and say, ‘Here’s what it’s good for’ only works to a degree. In the Q-Lab, users with their various applications are going to find out for themselves what these early-stage quantum computers are good for. Having a diverse user base is going to be very helpful.”
What’s in it for the company? With its enormous, broad-ranging quantum enterprise, UMD is a knowledge base and resource for chasing wild new ideas that a laser-focused tech company can't afford in-house, said David Steuerman, IonQ director of academic and national lab partnerships.
“Any application we want to explore, we can knock on a door and say, ‘Hey, come on over to the Q-Lab and work with us, because we want to work with you,’” he said. “We have great hardware, so we think they’ll want to work with us.”
Perhaps one of the facility’s grandest features will be a node connecting IonQ to a pioneering quantum network—the first step toward a quantum internet—under development at the QTC in an NSF-funded project led by engineering and physics Professor Edo Waks. Said Walsworth, “The plan is to make this the largest, longest-distance quantum network in the world”—filling in another broad swath of white space in the map of the quantum future.
Original news story written by Chris Carroll